Understanding the Thermodynamic Properties of Black Holes
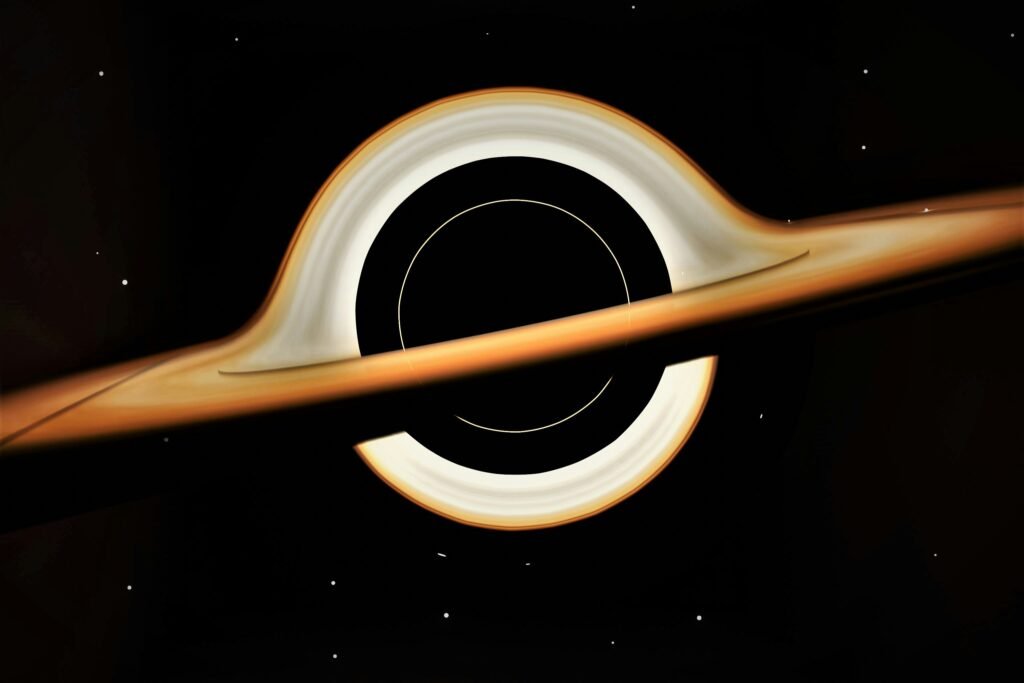
Introduction to Black Holes and Thermodynamics
Black holes are one of the most intriguing objects in the universe, attracting significant attention from both astronomers and physicists. Defined as regions in space where gravitational forces are so overwhelming that nothing, not even light, can escape their grasp, black holes challenge our understanding of physics. They generally form from the remnants of massive stars that undergo gravitational collapse at the end of their life cycles. The resulting structure is characterized by an event horizon, a boundary beyond which escape is impossible, and a singularity, where matter is thought to be infinitely dense.
The study of thermodynamics, which deals with heat, work, and the laws governing energy transformation, has become increasingly relevant when discussing black holes. These celestial phenomena seem to possess entropy, a core concept in thermodynamic principles, indicating that they might obey the same statistical laws that govern other thermodynamic systems. One of the pivotal concepts is that the entropy of a black hole is proportional to the area of its event horizon, rather than its volume, marking a significant shift in how we understand physical laws at cosmic scales.
The historical relationship between black holes and thermodynamics was revolutionized by the work of several notable physicists, most famously Stephen Hawking. In the 1970s, Hawking’s theoretical insights led to the conclusion that black holes are not entirely black; they emit radiation, now known as Hawking radiation, due to quantum mechanical effects near the event horizon. This breakthrough merged classical mechanics with quantum mechanics, creating pathways for scientists to explore connections between gravitational phenomena and thermodynamic laws. Understanding these relationships is essential for advancing our knowledge in astrophysics and confronting questions about the universe’s origins and ultimate fate.
The Laws of Black Hole Thermodynamics
The study of black holes has led to the formulation of four distinct laws that parallel the principles of classical thermodynamics. These laws serve not only as theoretical constructs but also as fundamental guidelines that describe the behavior of black holes in relation to thermodynamic processes. Each law sheds light on the unique characteristics of these enigmatic celestial bodies.
The first of these laws, often referred to as the zeroth law of black hole thermodynamics, asserts that black holes have a uniform temperature across their event horizons. This can be likened to how temperature is uniform in a system at thermal equilibrium in classical thermodynamics. The uniformity refers to the fact that, regardless of size or mass, a black hole emits Hawking radiation at a constant temperature determined solely by its mass. The implications of this phenomenon are profound, as it indicates that black holes do not merely embody singularities but also possess thermodynamic properties akin to traditional physical systems.
The first law pertains to the conservation of energy within a black hole system. This law states that the change in the mass of a black hole corresponds directly to the thermal energy emitted and the work done on or by the black hole. It can be summarized as a relationship between variations in mass, temperature, and entropy. Following this, the second law posits that the entropy of a black hole is proportional to the area of its event horizon, rather than its volume. This finding intertwines black hole physics with the concept of entropy, suggesting that the total entropy of a system, including that of black holes, should never decrease.
Lastly, the third law states that it is impossible to reduce the temperature of a black hole to absolute zero. This aligns with the classical assertion that systems cannot reach absolute zero due to the Heisenberg uncertainty principle, which prevents a system from achieving a state of minimal entropy. Together, these laws collectively establish a framework for understanding black hole thermodynamics, enriching our comprehension of these complex entities despite their unique properties.
Entropy and Information Paradox
Entropy, a fundamental concept in thermodynamics, measures the degree of disorder within a system. In the realm of black holes, entropy takes on a unique significance. According to the laws of thermodynamics, particularly the second law, entropy tends to increase as energy becomes more dispersed. For black holes, this principle is intriguingly linked to their surface area, as articulated by Jacob Bekenstein and later expanded upon by Stephen Hawking. The Bekenstein-Hawking formula proposes that a black hole’s entropy is proportional to the area of its event horizon, presenting the surprising finding that a black hole can encapsulate a vast amount of information within its surface area, despite its seemingly simplistic nature.
This leads to the famous black hole information paradox, which challenges our understanding of physics. When matter crosses the event horizon, it seemingly disappears from the observable universe, raising the question: what happens to the information contained within that matter? Hawking’s initial proposal suggested that information is lost forever when a black hole evaporates, a notion that directly contrasts with the principles of quantum mechanics, which maintain that information cannot be destroyed. This paradox has sparked extensive debate among physicists, leading to multiple theories regarding the fate of information.
One prominent interpretation is the concept of “information scrambling,” which implies that information is not lost but rather transformed and spread throughout the black hole, ultimately being released during Hawking radiation. Alternatively, some theorists posited that information may be stored in a two-dimensional form on the event horizon, known as holographic principles. These discussions underscore the intricate relationship between entropy, information, and the fundamental laws governing our universe.
As research continues, the implications of black hole entropy may extend beyond astrophysics, influencing our comprehension of information theory and quantum mechanics. This ongoing exploration signifies a monumental endeavor in understanding the universe’s most enigmatic phenomena.
Implications and Future Research in Black Hole Thermodynamics
The study of black hole thermodynamics has far-reaching implications for modern physics, bridging concepts from quantum gravity, quantum field theory, and cosmology. Black holes challenge our understanding of fundamental principles, leading to profound insights into the interplay between spacetime and thermodynamics. For instance, the identification of temperature and entropy associated with black holes has prompted theorists to rethink the laws of thermodynamics. These insights encourage further investigation into how black holes function within the broader framework of the universe.
Ongoing research into Hawking radiation, proposed by physicist Stephen Hawking, exemplifies the efforts to demystify black holes. Hawking radiation implies that black holes are not entirely black; they emit thermal radiation due to quantum effects near the event horizon. This phenomenon has substantial implications for quantum field theory and prompts critical questions regarding the fate of information swallowed by black holes. Addressing these questions could bridge the gap between quantum mechanics and general relativity, thus leading to a more unified understanding of physics.
Moreover, the exploration of black holes may provide insights into the fundamental laws of the universe. Observations of accretion disks and gravitational waves from merging black holes offer empirical data that can be reconciled with theoretical predictions. Such studies may illuminate the role of black holes in cosmic evolution, including galaxy formation and the distribution of matter throughout the universe.
As research progresses, potential discoveries related to black hole thermodynamics could reshape our understanding of the cosmos, shifting paradigms in both theoretical and observational astrophysics. The implications of these findings extend beyond black holes, prompting inquiries into dark energy and the fabric of spacetime itself. Hence, the future of black hole research not only enhances our comprehension of these enigmatic entities but also advances our grasp of the universe at large.